GHG emissions from beef and sheep production are a very significant source of GHGs from UK agriculture.
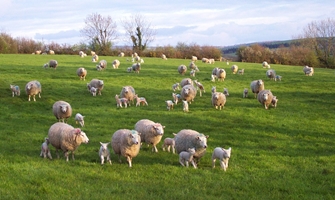
Of the UK’s total agricultural area (18,819 hectares) grassland contributes the largest proportion with approximately 11,345 hectares of land classified as rough grazing, temporary or permanent pasture in 2020. There is therefore clearly a role for beef and sheep production in the UK to utilise this substantial land area that is unsuitable for cropping. In regards to GHG emissions, reduced stocking rates would immediately reduce the farm’s overall GHG output and consequent CO2e footprint, but may not be the best approach for business security, land-use or habitat safeguarding. Furthermore, there must be consideration for the emission contribution from importing meat products which if are not produced in the UK must be sourced from elsewhere.
The majority of GHGs from beef and sheep are emitted as methane (CH4) – this is a by product of the ruminant’s digestive process as it converts forage to useful energy. Another significant GHG emission is nitrous oxide (N20) which is emitted mainly as a result of fertiliser and manure applications in the process of producing food and bedding used as part of the wider livestock production process. Data collected by the FCT suggest that often the use of diesel, electricity and direct carbon dioxide (CO2) emissions are less than 10% of a total farm carbon footprint, so in comparison to the afore mentioned emission sources are of lower priority.
Mitigation Strategies:
Improving Overall Efficiencies of the Flock/Herd:
This is the route recommended by the major industry organisations and, where possible to implement, will benefit the farm business as well as reducing farm GHG emissions.
The Scottish Agricultural College have put numbers to a couple of immediate efficiency indicators in beef production systems:
- Reducing calf mortality rates by 5% will reduce GHG emissions by 10% per kg carcass weight.
- Increasing the energy content of ensiled grass by 1MJ/kg DM will improve feed quality and DM intakes resulting in a reduction of GHGs by approximately 6% per kg of carcass weight.
- Teagasc, the Irish research and extension service, estimate that there are differences of over 3x between the ‘worst’ and the ‘best’ beef farms across the range of production systems. They calculate that an extension in the grazing period by two weeks either side of housing cattle can reduce GHG emissions by over 5% due primarily to the reduction of slurry stored and the higher digestibility of grazed grass compared to conserved forage resulting in better live weight gains. They are currently working on a ‘carbon navigator’ tool that will be used by extension workers to assess current KPIs and plot improved efficiency targets for farms to aim at that can be quantified.
- Improved health status, improved feed conversion efficiency and any management changes to increase live weight gain will have corresponding benefits to all aspects of the farm business.
- The opportunities for diet modification are far less than with dairy herds as nearly all beef and sheep are extensively reared with little concentrate inputs. However incorporating legumes (and where possible high sugar rye grasses to increase the feed value of the grass will also benefit both bottom lines.
- The area for greatest GHG reductions is around the application of manures and fertilisers. Any reduction in the use of fertilisers will have a significant effect as N2O is the most potent of all GHGs. Careful application techniques for manures and careful timing of all applications to avoid putting on more nutrients than can be taken up by plant growth or taken into the soil organic matter will significantly reduce GHG emissions. It is equally important to not apply nutrients to waterlogged soils as N2O emissions will be very high in these conditions – and you’re losing nearly all the Nitrogen that you’re putting on to feed the pasture.
Replacing Nitrogen Fertilisers with Legumes:
Legumes such as clover have the ability to fix nitrogen and can replace or reduce the need to apply artificial nitrogen. Introducing clovers, or other legumes, into established permanent pasture requires attention to detail but is relatively straightforward to achieve, and, once established they can produce similar amounts of forage to fertilized grass without the cost or GHG emissions.
Manure Storage:
There are a number of studies on how different storage techniques for solids and slurries affect GHG emissions with conflicting results.
Methane producing bacteria thrive in anaerobic conditions and higher temperatures therefore aerating slurry stores, regularly turning muck heaps and emptying the slurry/manure store before the summer will reduce CH4 emissions. However ammonia and nitrous oxide losses are generally lower in aerated conditions – one study found losses of N2O to be half from aerated slurry stores than un-aerated stores. It is agreed by all researchers that using a trailing shoe for slurry applications reduces GHG emissions (and nitrogen losses) as immediate soil contact reduces losses of ammonia with consequent reduction in N2O emissions.
Mob stocking:
Mob stocking is a technique that is being developed in many countries as an alternative pasture management system for beef. It runs counter to the conventional grazing strategies which encourage regular and frequent grazing. In mob stocking the intervals between grazing are 2-3x as long, with the grass much more matured when grazed. The cattle are only on any area of grass for a few days maximum, at very high densities, and trample a significant proportion of the grass into the soil. This ‘wasted’ grass increases soil organic matter content with a corresponding sequestration of carbon. See soil sequestration section for more details.
Further reading:
To find out how to account for sheep in your enterprise head here.
Recent Comments